This week’s Shortwave Radiogram was an interesting test of digital mode in a variety of propagation conditions. Many times, the Radiogram is transmitted using MFSK modes. This week, with Springtime coming in and propagation changes happening, Olivia 64-2000 was used to transmit a portion of the program.
With this experiment happening, I took advantage of two transmit periods that were well outside what I would consider appropriate frequencies for the time of day and the distance from the transmitter. The first reception was made on Thursday at 2330 on 9265kHz from WINB in Pennsylvania. As expected, the transmission was not received 100%. In fact, the only portion that was received complete was the part transmitted in Olivia 64-2000.
The second reception was made on Saturday at 1330 on 15770kHz from WRMI in Florida. As the first reception, it was received broken with the exception of the Olivia 64-2000 portion.
My usual sked of 2330 on 7780kHz from WRMI Florida was received really well, as usual.
The audio, as received at my station, is available for download if you’d like to try to decode it yourself.
Welcome to program 146 of Shortwave Radiogram. I'm Kim Andrew Elliott in Arlington, Virginia USA. Here is the lineup for today's program, in modes as noted: 1:50 MFSK32: Program preview (now) 3:07 Olivia 64-2000**: Seismic impact of stay-at-home 7:41 MFSK16: Seismic impact continued 10:24 MFSK64: Harvesting energey from Wi-Fi 16:14 This week's images* 27:48 MFSK32: Closing announcements * with images ** Turn off Fldigi squelch (SQL) and choose receiver bandwidth that will accept the data at 500 to 2500 Hz above and/or below the carrier frequency. Please send reception reports to radiogram@verizon.net And visit http://swradiogram.net Twitter: @SWRadiogram Shortwave Radiogram now changes to Olivia 64-2000 ... Before RSID: <<2020-04-05T23:33Z MFSK-32 @ 1422100+1499>> This is Shortwave Radiogram in Olivia 64-2000 Scientists Report Coronavirus Shutdowns Have Reduced Seismic 'Noise' VOA News 31 March 2020 Researchers who study Earth's movements say mandatory shutdowns of transportation systems and other human activities as a result of the coronavirus pandemic has resulted in a drop in what they call seismic "noise" around the world. An article published Tuesday in the scientific research journal Nature explains that human activity, such as moving vehicles and industrial machinery, can move Earth's crust the way earthquakes and volcanic activity do. And researchers say the lack of such human activity in recent days has made a significant difference. Article continues in M Before RSID: <<2020-04-05T23:37Z OL 64-2K @ 1422100+1504>> This is Shortwave Radiogram in MFSK16 Royal 7dcD ory of Belgium seismologist Thomas Lecocq says vibrations caused by human activity have dropped by one-third since coronavirus containment measures were introduced in that country. Researchers at the California Institute of Technology reported a similar drop in the Los Angeles area, as did researchers in Britain. Nature reports the reduced human-generate "noise" has allowed scientists to get more accurate and sensitive readings regarding earthquake aftershocks in urban areas that might otherwise go undetected. The researchers say this also allows for the study of more subtle vibrations, such as those generated by ocean waves crashing, which help when probing the Earth’s crust. bit.ly/2UxmUSO Changing to MFSK64 ... Before RSID: <<2020-04-05T23:40Z MFSK-16 @ 1422100+1499>> This is Shortwave Radiogram in MFSK64 Please send your reception report to radiogram@verizon.net From MIT News: Energy-harvesting design aims to turn Wi-Fi signals into usable power Device for harnessing terahertz radiation might enable self-powering implants, cellphones, other portable electronics. Jennifer Chu 27 March 2020 Any device that sends out a Wi-Fi signal also emits terahertz waves —electromagnetic waves with a frequency somewhere between microwaves and infrared light. These high-frequency radiation waves, known as "T-rays," are also produced by almost anything that registers a temperature, including our own bodies and the inanimate objects around us. Terahertz waves are pervasive in our daily lives, and if harnessed, their concentrated power could potentially serve as an alternate energy source. Imagine, for instance, a cellphone add-on that passively soaks up ambient T-rays and uses their energy to charge your phone. However, to date, terahertz waves are wasted energy, as there has been no practical way to capture and convert them into any usable form. Now physicists at MIT have come up with a blueprint for a device they believe would be able to convert ambient terahertz waves into a direct current, a form of electricity that powers many household electronics. Their design takes advantage of the quantum mechanical, or atomic behavior of the carbon material graphene. They found that by combining graphene with another material, in this case, boron nitride, the electrons in graphene should skew their motion toward a common direction. Any incoming terahertz waves should "shuttle" graphene's electrons, like so many tiny air traffic controllers, to flow through the material in a single direction, as a direct current. The researchers have published their results today in the journal Science Advances, and are working with experimentalists to turn their design into a physical device. "We are surrounded by electromagnetic waves in the terahertz range," says lead author Hiroki Isobe, a postdoc in MIT's Materials Research Laboratory. "If we can convert that energy into an energy source we can use for daily life, that would help to address the energy challenges we are facing right now." Isobe's co-authors are Liang Fu, the Lawrence C. and Sarah W. Biedenharn Career Development Associate Professor of Physics at MIT; and Su-yang Xu, a former MIT postdoc who is now an assistant professor chemistry at Harvard University. Breaking graphene's symmetry Over the last decade, scientists have looked for ways to harvest and convert ambient energy into usable electrical energy. They have done so mainly through rectifiers, devices that are designed to convert electromagnetic waves from their oscillating (alternating) current to direct current. Most rectifiers are designed to convert low-frequency waves such as radio waves, using an electrical circuit with diodes to generate an electric field that can steer radio waves through the device as a DC current. These rectifiers only work up to a certain frequency, and have not been able to accommodate the terahertz range. A few experimental technologies that have been able to convert terahertz waves into DC current do so only at ultracold temperatures — setups that would be difficult to implement in practical applications. Instead of turning electromagnetic waves into a DC current by applying an external electric field in a device, Isobe wondered whether, at a quantum mechanical level, a material's own electrons could be induced to flow in one direction, in order to steer incoming terahertz waves into a DC current. Such a material would have to be very clean, or free of impurities, in order for the electrons in the material to flow through without scattering off irregularities in the material. Graphene, he found, was the ideal starting material. To direct graphene's electrons to flow in one direction, he would have to break the material's inherent symmetry, or what physicists call "inversion." Normally, graphene's electrons feel an equal force between them, meaning that any incoming energy would scatter the electrons in all directions, symmetrically. Isobe looked for ways to break graphene's inversion and induce an asymmetric flow of electrons in response to incoming energy. Looking through the literature, he found that others had experimented with graphene by placing it atop a layer of boron nitride, a similar honeycomb lattice made of two types of atoms — boron and nitrogen. They found that in this arrangement, the forces between graphene's electrons were knocked out of balance: Electrons closer to boron felt a certain force while electrons closer to nitrogen experienced a different pull. The overall effect was what physicists call "skew scattering," in which clouds of electrons skew their motion in one direction. Isobe developed a systematic theoretical study of all the ways electrons in graphene might scatter in combination with an underlying substrate such as boron nitride, and how this electron scattering would affect any incoming electromagnetic waves, particularly in the terahertz frequency range. He found that electrons were driven by incoming terahertz waves to skew in one direction, and this skew motion generates a DC current, if graphene were relatively pure. If too many impurities did exist in graphene, they would act as obstacles in the path of electron clouds, causing these clouds to scatter in all directions, rather than moving as one. "With many impurities, this skewed motion just ends up oscillating, and any incoming terahertz energy is lost through this oscillation," Isobe explains. "So we want a clean sample to effectively get a skewed motion." One direction They also found that the stronger the incoming terahertz energy, the more of that energy a device can convert to DC current. This means that any device that converts T-rays should also include a way to concentrate those waves before they enter the device. With all this in mind, the researchers drew up a blueprint for a terahertz rectifier that consists of a small square of graphene that sits atop a layer of boron nitride and is sandwiched within an antenna that would collect and concentrate ambient terahertz radiation, boosting its signal enough to convert it into a DC current. "This would work very much like a solar cell, except for a different frequency range, to passively collect and convert ambient energy," Fu says. The team has filed a patent for the new "high-frequency rectification" design, and the researchers are working with experimental physicists at MIT to develop a physical device based on their design, which should be able to work at room temperature, versus the ultracold temperatures required for previous terahertz rectifiers and detectors. "If a device works at room temperature, we can use it for many portable applications," Isobe says. He envisions that, in the near future, terahertz rectifiers may be used, for instance, to wirelessly power implants in a patient's body, without requiring surgery to change an implant's batteries. Such devices could also convert ambient Wi-Fi signals to charge up personal electronics such as laptops and cellphones. "We are taking a quantum material with some asymmetry at the atomic scale, that can now be utilized, which opens up a lot of possibilities," Fu says. This research was funded in part by the U.S. Army Research Laboratory and the U.S. Army Research O?ce through the Institute for Soldier Nanotechnologies (ISN). http://news.mit.edu/2020/energy-harvesting-wi-fi-power-0327 This is Shortwave Radiogram in MFSK64 Please send your reception report to radiogram@verizon.net This week's images ...
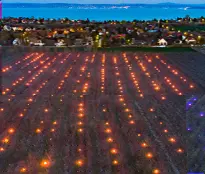
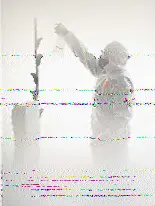
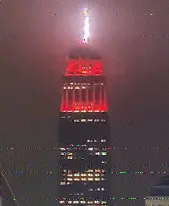
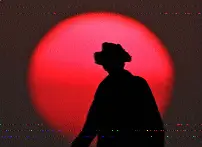
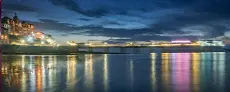
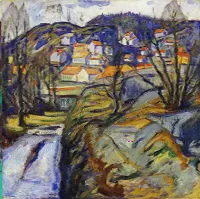
Shortwave Radiogram returns to MFSK32 ... Before RSID: <<2020-04-05T23:58Z MFSK-64 @ 1422100+1499>> This is Shortwave Radiogram in MFSK32 ... Shortwave Radiogram is transmitted by: WRMI, Radio Miami International, wrmi.net and WINB Shortwave, winb.com Please send reception reports to radiogram@verizon.net And visit http://swradiogram.net Twitter: @SWRadiogram or twitter.com/swradiogram I'm Kim Elliott. Please join us for the next Shortwave Radiogram.